Team:Cambridge/Project
From 2011.igem.org
Felix Zhou (Talk | contribs) (→Light and interference - The physics behind structural colour) |
Felix Zhou (Talk | contribs) (→Light and interference - The physics behind structural colour) |
||
Line 31: | Line 31: | ||
[[File:Cam_ThinFilmInterference.jpg | thumb | Diagram showing how light reflects from a thin film]] | [[File:Cam_ThinFilmInterference.jpg | thumb | Diagram showing how light reflects from a thin film]] | ||
- | The difference in [http://en.wikipedia.org/wiki/Refractive_index refractive index] between the alternating layers leads to reflection (bouncing off) and transmission of light. This process is repeated at each layer. When the two reflected light waves meet, they sum together but are no longer ''in sync'' due to differences in optical paths leading to the phenomenon known as interference. [[File:Cam-intereference_two_ waves.png | thumb | '''Left:''' Constructive interference | + | The difference in [http://en.wikipedia.org/wiki/Refractive_index refractive index] between the alternating layers leads to reflection (bouncing off) and transmission of light. This process is repeated at each layer. When the two reflected light waves meet, they sum together but are no longer ''in sync'' due to differences in optical paths leading to the phenomenon known as interference. [[File:Cam-intereference_two_ waves.png | thumb | '''Left:''' Constructive interference '''Right:''' Destructive interference]][[File:Cam-2D_interference.png | thumb | Examples of 2D interference patterns]] Some wavelengths will destructively interfere more than others. The result is that not all colours are reflected with the same intensity rainbow-like effects like those of oil droplets on the surface of water or the surface of soap films which 'shimmer'. |
Revision as of 09:52, 3 August 2011
Contents |
Background to our project
How to disappear completely - Reflectins in cephalopods
The beautiful optical properties which reflectins make possible are used for different purposes in nature. Manipulation of reflectance may allow squid to communicate through polarised light. Altering their refractive index to match that of the water column allows cephalopods to hide from their predators - a living invisibility cloak.
Reflectin was first identified as such in the Hawaiian bobtail squid [http://en.wikipedia.org/wiki/Euprymna_scolopes Euprymna scolopes] as the protein responsible for a reflective layer in the "light organ". This allows light emitted by symbiotic bacteria to be reflected downwards away from the squid, like a [http://en.wikipedia.org/wiki/Headlamp#Reflector_lamps car headlamp].
The Longfin inshore squid [http://en.wikipedia.org/wiki/Longfin_Inshore_Squid Loligo pealei] shows dynamic iridescence controlled through signals from the nervous system[5] which turn on a [http://en.wikipedia.org/wiki/Kinase protein kinase], an enzyme which adds negatively charged phosphate groups to the positively charged protein. This alters the attraction/repulsion between the platelet arrangement of reflectins, changing the spacing of the iridophore layers and therefore the colour of light reflected. (Read more about structural colour)
Squid skin contains multiple specialised cell types designed to allow highly controlled changes of colour and reflectance to optimise camouflage. The beautiful optical properties in light reflecting tissues occur due to a hierarchy of structural arrangements - the structure of reflectin protein itself, the complex platelets which the protein forms, and the shape and layering of reflective cells. A thin tissue layer made up of iridocytes close to the surface of squid skin is made up of ~40% reflectin (out of the total protein content)[2]. Within iridocytes, reflectins self assemble to form membrane associated platelets. The changes in refractive index as light moves through the layers of reflectin and cytoplasm forms a natural Bragg reflector[3].
EM of reflectin ultrastructure (dark bands) in cells from Loligo opalescens[6] |
EM of reflectin ultrastructure (dark bands) in reflector cells from Octopus dofleini[6] |
Iridophores which appear similar in structure to those found in cephalopods are also seen in other members of mollusc phylum - giant clams[4]. They are responsible for the stunning iridescent colours of the mantle, and may play a role in protecting against harmful UV and maximising capture of sunlight for the photosynthetic symbionts which live alongside the clam.
Light and interference - The physics behind structural colour
Structural colour is a common occurrence in nature - butterfly wings, fish scales and even [http://en.wikipedia.org/wiki/Tapetum_lucidum cats's eyes] contain light reflecting components rather than pigments. It occurs due to the phenomenon of [http://en.wikipedia.org/wiki/Thin-film_interference thin film interference].
The difference in [http://en.wikipedia.org/wiki/Refractive_index refractive index] between the alternating layers leads to reflection (bouncing off) and transmission of light. This process is repeated at each layer. When the two reflected light waves meet, they sum together but are no longer in sync due to differences in optical paths leading to the phenomenon known as interference. Some wavelengths will destructively interfere more than others. The result is that not all colours are reflected with the same intensity rainbow-like effects like those of oil droplets on the surface of water or the surface of soap films which 'shimmer'.
The observed colour changes with viewing angle, angle of incidence of light and alterations in the spacing of the layers or refractive indices which alters the mechanism of interference. In fact by matching the refractive indices of individual layers total transparency and hence invisibility can be achieved!
This principle of interference is believed to be the primary mechanism by which colour is controlled in the skin of squid. Experimental observations of changes in the thickness and spacing between reflectin layers in vivo has been observed following post translational modification.
Reflectins as novel polymers
Reflectins have unique properties in part due to their unique amino acid composition - residues which are normally common in proteins (alanine, isoleucine, leucine and lysine) are nowhere to be seen in any reflectins identified so far, whilst typically rare residues (arginine, methionine, tryptophan and tyrosine) make up ~57% of the protein. The family of reflectin proteins share a repeated domain which may also possess unique optical properties.
The 3D protein structure of reflectin has not yet been characterised. It may be natively unstructured - Weiss et al hypothesised that it may form a [http://en.wikipedia.org/wiki/Beta_barrel beta barrel]-like structure when interacting with membranes, as recombinant reflectin-like proteins associated strongly with artificial membrane structures after cell-free expression.
Kramer et al and Tao and DeMartini et al have demonstrated the remarkable capability of reflectin proteins to self assemble in vitro to create complex structures. Recombinant reflectin, refolded in vitro, can be carefully spread along a silicon slide to make thin films with intense structural colours from thin film interference. Measuring the refractive index of this in vitro arrangement of the protein reveals that it possesses the highest refractive index of any known protein. Kramer et al also demonstrated the ability of reflectin to form a diffraction grating when the ionic solvents used to dissolve it were diffused away in a water bath. This ultrastructural arrangement showed iridescence.
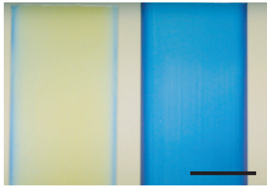
References
[http://www.nature.com/nmat/journal/v6/n7/abs/nmat1930.html] Kramer et al. The self-organizing properties of squid reflectin protein Nature Materials 533-538 VOL6 JULY 2007 [http://www.sciencemag.org/content/303/5655/235.short] Crookes et al. Reflectins: The Unusual Proteins of Squid Reflective Tissues SCIENCE 235-238 VOL303 9 JANUARY 2004 [http://www.sciencedirect.com/science/article/pii/S0142961209011442] Morse et al. The role of protein assembly in dynamically tunable bio-optical tissues Biomaterials 793-801 VOL31 FEBRUARY 2010 [http://www.publish.csiro.au/paper/ZO9920319.html] Griffiths et al. Iridophores in the mantle of giant clams. Australian Journal of Zoology (1992) Volume: 40, Issue: 3 Pages: 319-326 [http://www.ncbi.nlm.nih.gov/pubmed/19776150] Izumi et al. Changes in reflectin protein phosphorylation are associated with dynamic iridescence in squid. J. R. Soc. Interface 6 March 2010 vol. 7 no. 44 549-560 [http://www.springerlink.com/content/bba14b73ad35f495/]Brocco et al. Reflector cells in the skin of Octopus dofleini Cell and Tissue Research, Volume 205, Number 2, 167-186, 1980