Team:ETH Zurich/Modeling
From 2011.igem.org
(→Roundup) |
(→Roundup) |
||
Line 11: | Line 11: | ||
== Roundup== | == Roundup== | ||
[[File:ETH codeExample.png|350px|left|thumb|Most of the modeling was done in Matlab. The 3D diffusion models were implemented using COMSOL.]] | [[File:ETH codeExample.png|350px|left|thumb|Most of the modeling was done in Matlab. The 3D diffusion models were implemented using COMSOL.]] | ||
- | Our goal was to create a large, spatiotemporal 3D reaction-diffusion model not only reliably reproducing the molecular dynamics of a single cell, but also the population dynamics arising from the intra-cell communication with acetaldehyde, xylene and AHL. Since the gradients of | + | Our goal was to create a large, spatiotemporal 3D reaction-diffusion model not only reliably reproducing the molecular dynamics of a single cell, but also the population dynamics arising from the intra-cell communication with acetaldehyde, xylene and AHL. Since the gradients of these signaling molecules are not only sensed, but also created by the SmoColi cells in a cooperative manner, a single cell model alone would not have been able to fully capture the dynamics of the microfluidic cellular sensor device. |
- | + | Nevertheless, we started our modeling efforts in designing a single cell model based on ordinary differential equations. Although this single cell model can not answer any questions regarding the formation of the acetaldehyde, xylene and AHL gradients, it can be reliably used to predict if the sensor, band-pass filter and alarm system works, given that there is a gradient of the signaling molecules. | |
- | + | In parallel to this effort we created a three dimensional dynamic reaction-diffusion model using the mechanical engineering software platform COMSOL. Different to the single cell model, this model can not provide any answers regarding the dynamics inside each cell. However, it can give information on if a gradient of acetaldehyde or xylene can be obtained, given that the degradation rate of these molecules per cell does not depend on the state of the rest of the synthetic network. | |
- | + | With these two separate models most of the questions regarding biological implementation and channel design could already be answered. However, the AHL alarm system depends neither only on the inter-cellular, nor only on the extra-cellular state of the overall network, but on both. Therefore, we combined both models in a final step to create a three dimensional, temporal, molecular reaction diffusion model, which can reliably reproduce all important characteristics of our SmoColi smoke-detector. | |
- | + | These modeling efforts were not only valuable in the decision process on how to construct the overall network, but also played a crucial role in the construction process of our microfluidic device. | |
|} | |} |
Revision as of 02:08, 22 September 2011

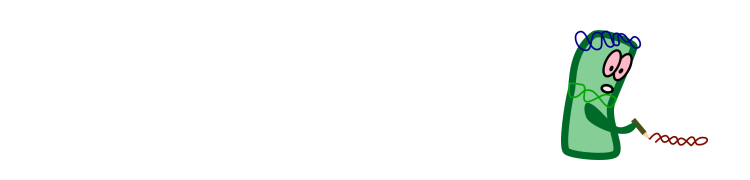
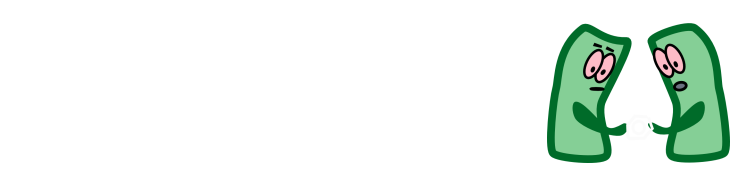


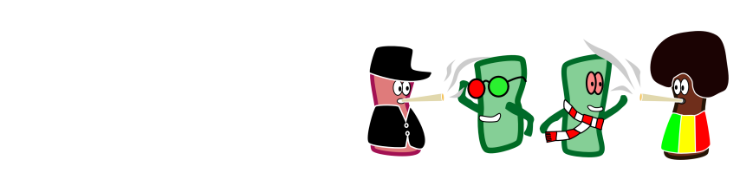
Modeling Overview | |
We created a computational model of our system in order to check whether our ideas might work in reality. We investigated questions such as for which range of acetaldehyde input we get a GFP band from the band-pass filter, or what the most suitable channel dimensions are in silico. Only afterwards we started with the actual experimental channel design. |
RoundupOur goal was to create a large, spatiotemporal 3D reaction-diffusion model not only reliably reproducing the molecular dynamics of a single cell, but also the population dynamics arising from the intra-cell communication with acetaldehyde, xylene and AHL. Since the gradients of these signaling molecules are not only sensed, but also created by the SmoColi cells in a cooperative manner, a single cell model alone would not have been able to fully capture the dynamics of the microfluidic cellular sensor device. Nevertheless, we started our modeling efforts in designing a single cell model based on ordinary differential equations. Although this single cell model can not answer any questions regarding the formation of the acetaldehyde, xylene and AHL gradients, it can be reliably used to predict if the sensor, band-pass filter and alarm system works, given that there is a gradient of the signaling molecules. In parallel to this effort we created a three dimensional dynamic reaction-diffusion model using the mechanical engineering software platform COMSOL. Different to the single cell model, this model can not provide any answers regarding the dynamics inside each cell. However, it can give information on if a gradient of acetaldehyde or xylene can be obtained, given that the degradation rate of these molecules per cell does not depend on the state of the rest of the synthetic network. With these two separate models most of the questions regarding biological implementation and channel design could already be answered. However, the AHL alarm system depends neither only on the inter-cellular, nor only on the extra-cellular state of the overall network, but on both. Therefore, we combined both models in a final step to create a three dimensional, temporal, molecular reaction diffusion model, which can reliably reproduce all important characteristics of our SmoColi smoke-detector. These modeling efforts were not only valuable in the decision process on how to construct the overall network, but also played a crucial role in the construction process of our microfluidic device. |